Message in a bottle: Open source technology to track the movement of plastic pollution
By Meagan Ando, SRC intern
The oceans on our planet are intricate, expansive, and provide a home for many organisms while maintaining a delicate balance that makes this environment so inhabitable. However, in recent times, many anthropogenic effects have been threatening them, one of which is the infamous plastic water bottle. Single-use plastic water bottles are all too familiar and are now so common that a majority of people would say they’ve seen one at some point while in natural areas. Based on beach clean-ups performed over 25 years, the International Coastal Cleanup listed these single-use nightmares fifth on the list of reported items of marine pollution (International Coastal Cleanup 2020). But how these pieces of trash end up in the oceans is a major topic of study for scientists worldwide. Because a large amount of debris is thought to originate from inland communities, rivers act as a critical transportation method as large quantities of garbage are dumped into the ocean and have been found to contribute to about 70-80% of all plastics present within the environment (Horton et al. 2017, Sarkar et al. 2019, Law and Thompson 2014). Previous studies have analyzed and modeled the drifting of this waste, which provided a basis for the research that Duncan et al. (2020) wanted to undertake. This group applied GPS networks and satellite technology to modified 500 mL plastic bottles deployed in the Ganges River and the Bay of Bengal. This technology was used due to its possibility to better understand movement through rivers and into marine habitats and how these quantities could potentially pollute open-ocean systems (Jambeck et al. 2015, Schmidt et al. 2017).
To carry out this study, replicated “bottle tags” were designed and built to replicate movement patterns of a plastic bottle based on size, shape, and buoyancy (Figure 1) (Duncan et al. 2020). Custom electronics were constructed using a computer-aided design model along with O-rings and epoxy to keep the device afloat. The tools were deployed during the National Geographic Sea to Source Ganges Expedition in 2 phases. Ten Phase A bottles were released in the pre-monsoon season and configured to activate every 3 hours to acquire a GPS fix, while a total of 15 Phase B bottles (12 in the river pre-monsoon and 3 in the bay) were deployed and programmed to mobilize every 4 hours to spend up to 30 seconds acquiring a GPS position before returning to a resting state until a satellite passed over (Duncan et al. 2020).
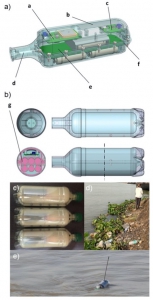
Figure 1: Visualization of the size, shape, and internal makeup of the deployment devices (Duncan et al. 2020).
Overall, the maximum distance tracked came out to be 2845 km over 94 days. Phase A tags were tracked for an average of 20.1 ± 5.7 days and, when plotted, showed a ‘stepwise’ movement (Figure 2 (a, b, c)). These deployments showed an issue with human interference and consequently being removed from the river due to high urbanization. The 12 Phase B bottles in the river were trailed for an average of 23.1 ± 9.3 days and also showed this ‘stepwise’ displacement (Figure 2 (d)). However, the 3 Phase B bottles deployed in the open-ocean were tracked for an average of 41.6 ± 26.7 days and ended up displaying a more continuous shift over the period of time (Figure 2 (e, f)). The biggest issue with these devices was due to high fishing pressure, in that they would constantly become entangled in fishing gear. To conclude, this proof of concept was successfully displayed in order to help understand how plastic waste such as water bottles is transported through the environment. The capacity for which this satellite technology can be used shows a significant ability to increase and gain new knowledge identifying various habitats associated with the accumulation of plastic debris, which could help conserve those under the most immediate threat of degradation.
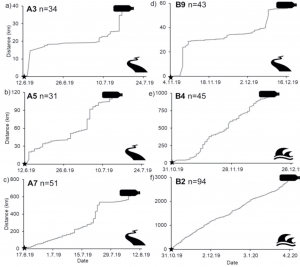
Figure 2: Bottle displacement versus time tracked (Duncan et al. 2020).
Works cited
Duncan EM, Davies A, Brooks A, Chowdhury GW, Godley BJ, Jambeck J, et al. (2020) Message in a bottle: Open source technology to track the movement of plastic pollution. PLoS ONE 15(12): e0242459.
Horton AA, Walton A, Spurgeon DJ, Lahive E, Svendsen C. Microplastics in freshwater and terrestrial environments: Evaluating the current understanding to identify the knowledge gaps and future research priorities. Sci Total Environ. 2017 May 15; 586:127–41
International Coastal Cleanup. Tracking Trash: 25 Years of Action for the Ocean (2020)
Jambeck JR, Geyer R, Wilcox C, Siegler TR, Perryman M, Andrady A, et al. Plastic waste inputs from land into the ocean. Science (80-). 2015 Feb 13; 347(6223):768–71.
Law KL, Thompson RC. Microplastics in the seas. Science (80-) [Internet]. 2014 Jul 11; 345(6193):144–5.
Sarkar DJ, Das Sarkar S, Das BK, Manna RK, Behera BK, Samanta S. Spatial distribution of meso and microplastics in the sediments of river Ganga at eastern India. Sci Total Environ. 2019 Dec 1; 694:133712.
Schmidt C, Krauth T, Wagner S. Export of Plastic Debris by Rivers into the Sea. Environ Sci Technol. 2017 Nov 7 ; 51(21):12246–53.